Intravesical Electrical Stimulation Improves Abnormal Prefrontal Brain Activity in Patients With Underactive Bladder: A Possible Central Mechanism
Article information
Abstract
Purpose
The aim of this study was to explore the mechanisms of central brain action in patients with neurogenic underactive bladder (UAB) treated with intravesical electrical stimulation (IVES).
Methods
We prospectively recruited patients with neurogenic UAB who chose to receive IVES treatment and healthy subjects (HS). At baseline, the following data were obtained: a 72-hour voiding diary; measurements of postvoid residual urine (PVR), voiding efficiency (VE) and first sensation of bladder filling (FS); American Urological Association Symptom Index Quality of Life (AUA-SI-QOL) scores, and functional near-infrared spectroscopy scans of the prefrontal cortex in the voiding stage. All UAB patients were re-evaluated for these indices after completing 4 weeks of IVES. A >50% improvement in PVR was defined as successful IVES treatment. Prefrontal activity was analyzed using the NIRS_KIT software, corrected with the false discovery rate (P<0.05). Statistical analysis was performed using IBM SPSS Statistics ver. 22.0, and P<0.05 was considered statistically significant.
Results
Eighteen UAB patients and 16 HS were included. IVES treatment was successful in 11 UAB patients and failed in 7. The PVR, VE, 24-hour clean intermittent catheterization, FS volume, and AUA-SI-QOL scores of the UAB group significantly improved after successful IVES treatment. BA9 (right dorsolateral prefrontal cortex [DLPFC]) and BA10 (right frontal pole) were significantly activated after successful IVES, and no significant difference was found between the successful group and HS group after IVES. Before IVES, BA10 (right frontal pole) was significantly deactivated in the failed group compared with the successful group.
Conclusions
The possible central mechanism of IVES treatment for neurogenic UAB is that IVES reactivates the right DLPFC and right frontal pole.
INTRODUCTION
Underactive bladder (UAB) is a symptomatic syndrome characterized by detrusor underactivity (DU), as observed in urodynamics [1,2]. The most recent symptomatic definition states, “UAB is characterized by a slow urinary stream, hesitancy and straining to void, with or without a feeling of incomplete bladder emptying and dribbling, often with storage symptoms” [3]. However, the International Continence Society has yet to provide a unified definition for UAB. The prevalence of UAB is notably high. Jeong et al. [4] reported that in a retrospective study, 40% of the men (254 out of 632) and 13% of the women (73 out of 547) were classified as having DU. Another study indicated that among patients with lower urinary tract dysfunction, the incidence of UAB was 38% (1,726 out of 4,538), with a distribution of 73% men and 27% women [5]. UAB has a significant impact on patients’ quality of life.
The treatment of UAB presents significant challenges, with the effectiveness and safety of its behavioral, pharmacological, and surgical interventions remaining subjects of debate [6,7]. Intravesical electrical stimulation (IVES) is a procedure that involves the insertion of a urinary catheter equipped with stimulating electrodes into the bladder. This technique utilizes normal saline as a conductive medium to stimulate the detrusor muscle, thereby activating the residual afferent nerve connections between the detrusor and the central nervous system. The goal is to induce a sensation of urinary urgency in the bladder, increase nerve impulse output, and ultimately enhance urination or improve urinary control [8,9]. As a form of conservative management, IVES is designed to improve bladder sensation, facilitate bladder emptying, and decrease postvoid residual urine (PVR) [8,9]. However, the precise mechanism by which IVES improves UAB has not been fully elucidated. IVES has been shown to directly activate Aδ afferent fibers from lowthreshold bladder mechanoreceptors, including the sensory systems responsible for initiating and maintaining voiding responses [10]. Some studies have posited that the voiding response induced by IVES is attributable to an increase in excitatory synaptic transmission within the central pathways that govern the voiding process [11]. One of our studies has suggested that IVES may exert its therapeutic effects on UAB peripherally by promoting the release of adenosine triphosphate from uroepithelial cells and concurrently inhibiting the release of nitric oxide from these cells [12]. Despite these findings, the specific mechanisms by which IVES affects the central nervous system in the treatment of neurogenic UAB remain to be determined.
Functional neuroimaging is crucial for research on the brain’s voiding pathways [13]. Imaging studies indicate that the prefrontal cortex (PFC) and anterior cingulate gyrus play a significant role in voiding, as evidenced by the activation of the right inferior frontal gyrus (near the dorsolateral frontal cortex) during this process [14]. Nour et al. [15] observed that cerebral blood flow in the superior frontal gyrus, middle frontal gyrus, and inferior frontal gyrus increased markedly during voiding in healthy subjects (HS). This was corroborated by urodynamic measurements taken concurrently with positron emission tomography scans, underscoring the PFC’s critical role in urination control. Functional near-infrared spectroscopy (fNIRS) is a noninvasive technique for imaging brain function that relies on optical principles. It offers several benefits: it is safe, noninvasive, and portable; it resists motion and electromagnetic interference; it provides high temporal and spatial resolution; and it allows for extended monitoring [16,17]. fNIRS is adaptable to any urination position and enables real-time tracking of brain activity during everyday urination. The accuracy and reliability of fNIRS signals have been confirmed by multiple studies, lending support to its application [16-18]. In this study, we used fNIRS to investigate real-time brain activity during the voiding stage in healthy adults and patients with UAB before and after IVES treatment. The aim was to elucidate the central brain mechanisms underlying the effectiveness of IVES in treating UAB.
MATERIALS AND METHODS
Subjects
With approval from the Institutional Review Board (IRB: 2021-085-1), we recruited 18 women with a mean age of 39.22±12.82 years diagnosed with neurogenic UAB who opted for IVES. Additionally, we enrolled 16 age- and sex-matched HS with a mean age of 39.88±12.54 years. All participants provided informed consent. The inclusion criteria for HS were as follows: (1) women aged between 18 and 60 years; (2) a normal 72-hour voiding diary; and (3) a urine volume of 200 to 400 mL per session, a free urine flow rate greater than 20 mL/sec, and a PVR volume measured by ultrasound of 50 mL or less. The exclusion criteria for HS included: (1) diseases related to the urinary system; (2) neurological system-related diseases; (3) major systemic diseases (e.g., cancer, heart, or respiratory diseases); (4) an inability to tolerate fNIRS; and (5) cognitive impairments that precluded cooperation.
The inclusion criteria for patients with UAB were as follows: (1) women between the ages of 18 and 60; (2) a diagnosis of UAB due to an incomplete spinal cord injury, with a disease duration of more than 3 months; (3) an American Spinal Injury Association grade of D or E; and (4) a history of using intermittent catheterization to empty the bladder. The indication for inclusion was a PVR volume exceeding 40% of the functional bladder capacity. The exclusion criteria for UAB patients were (1) low bladder compliance (less than 20 mL/cm H2O) as determined by urodynamic examination; (2) bladder outlet obstruction; (3) a symptomatic urinary tract infection; (4) hydronephrosis or vesicoureteral reflux; (5) renal insufficiency, defined as a serum creatinine level greater than 1.5 times the upper limit of normal; (6) malignant tumors of the bladder; (7) an implanted pacemaker or defibrillator; (8) dementia, cerebral atrophy, recent cerebrovascular disease, or cognitive impairment; (9) epilepsy or mental illness that could impede cooperation with medical staff; (10) positive results from preoperative infectious disease screening; (11) current pregnancy or intention to become pregnant in the near future; (12) participation in another clinical trial within the previous 3 months; and (13) any other conditions deemed relevant by the investigator.
Experimental Procedures
Subjects were not evaluated during menstruation. At baseline, all subjects completed a 72-hour voiding diary, underwent Bultrasonography-guided PVR and first sensation of bladder filling (FS) volume measurements, recorded their American Urological Association Symptom Index Quality of Life (AUA-SIQOL) scores, and participated in fNIRS of the PFC during voiding. Subsequently, patients with UAB received IVES treatments using an FES-D1 stimulator and a DJ-A01 stimulating electrode (General Stim, Inc., Hangzhou, Zhejiang, China) (Fig. 1) [19]. For the IVES procedure, a sterile, disposable 12F transurethral catheter with a DJ-A01 stimulating electrode was inserted into the bladder, which was then filled with 150 mL of body-temperature (37°C) sterile saline.
The reference patch electrode was positioned on the abdominal area just above the pubic bone. Both electrodes were connected to the FES-D1 stimulator. The stimulation parameters were set to a bipolar square wave pulse with a frequency of 10 Hz, a pulse width of 0.2 msec, and the maximum stimulus intensity that the patient could comfortably endure. Treatments were administered daily for 30 minutes, 5 days a week, over a period of 4 weeks. Treatment was halted immediately if any adverse events occurred, such as pain, intolerable itching, severe palpitations, or any other unbearable discomfort. Following IVES treatment, all patients with UAB were reassessed using the same baseline measures. The primary efficacy measure was PVR, while secondary efficacy measures included voiding efficiency (VE), the number of 24-hour clean intermittent catheterizations (CICs), FS volume, and the AUA-SI-QOL score. At baseline, PVR, VE, FS volume, and the number of 24-hour CICs were each evaluated three times consecutively, and the average of these measurements was taken as the baseline value for each index. These efficacy measures (PVR, VE, FS volume, and number of 24-hour CICs) were assessed in the same manner after 4 weeks of treatment. The AUA-SI-QOL was evaluated both at baseline and after the 4-week treatment period. VE was calculated using the formula: VE=(volume voided/[volume voided+PVR])×100%. A reduction in PVR of greater than 50% was considered a successful outcome of IVES treatment [20].
The fNIRS experiment was conducted as follows: (1) the subject’s urgency to urinate was assessed using the visual analog scale (0 to 10) when they experienced a strong physiological need [21]; (2) the subject was then helped to sit in the urination chair; (3) the fNIRS cap and electrodes were attached to the subject; (4) the room lights were dimmed, and the subject was instructed to close their eyes, remain silent and alert, and refrain from engaging in structured thinking; and (5) the subject was given a cue to start and another to stop urinating. The total duration of the scan was 180 seconds, with 60 seconds of prevoiding and 120 seconds during voiding (Fig. 2). Subjects were briefed on this procedure prior to each fNIRS scan.
fNIRS Equipment
To monitor the hemodynamic response in the PFC of subjects, we utilized a portable fNIRS system (Light-NIRS, Shimadzu Co., Kyoto, Japan). This system emits three wavelengths of continuous near-infrared light (780, 805, and 830 nm) through 16 optical fibers— comprising 8 light sources and 8 detectors—arranged in an alternating pattern on 2×8 matrix photoplates. These components form 22 detection channels, which are mounted on an elastic hat. The separation between each pair of emitter and detector fibers is approximately 3 cm, allowing for a detection depth of 2–3 cm beneath the scalp within the cerebral cortex [22]. Channels are formed by adjacent pairs of light-emitting electrodes and detectors (Fig. 3). The system’s signal collection frequency is set at 10 Hz. The probes are positioned 3 cm apart, with the lowest row aligned with the Fp1 to Fp2 line according to the international 10–20 system (Fig. 3) [23]. To record the spatial coordinates of all channels, we employed a 3-dimensional (3D) digitizer (Patriot, Polhemus, Colchester, VT, USA). We then used NIRS_SPM to estimate the Montreal Neurological Institute coordinates for each channel and to determine the probability of corresponding brain regions within the Brodmann area (BA) map [24]. The specific locations of the channels are detailed in Fig. 4.

The 2×8 array probe set (A) and 3D MNI coordinates of the 22 channels (B). 3D, 3‐dimensional; MNI, Montreal Neurological Institute; Ch, channel.
fNIRS Data Analysis
A MATLAB toolbox was used to perform data preprocessing and visualize the results [25]. Slow time drifts were removed by applying a first-order detrend [26]. For motion correction, we employed the temporal derivative distribution repair method [27]. To eliminate artifacts, the data were further filtered within a bandpass range of 0.001 to 0.08 Hz [28]. We concentrated on the oxyhemoglobin (oxy-Hb) signal due to its heightened sensitivity to local cerebral blood flow fluctuations [29].
We also carried out a channel-based, group-level analysis of the preprocessed fNIRS data. Initially, individual-level statistical analyses were performed using general linear models, and subtractive controls were employed to derive contrast values for each channel. Subsequently, at the group level, we applied the one-sample t-test on the individual-level contrast values to pinpoint channels that were activated during voiding. For intragroup data analysis within the UAB group, the paired t-test was utilized. Conversely, to analyze intergroup data between the HS and UAB groups, the independent-sample t-test was conducted. A P-value of less than 0.05 with false discovery rate (FDR) correction was deemed to indicate statistical significance. The results were graphically represented using the NIRS_KIT professional software.
Statistical Analysis
In this study, data processing was conducted using IBM SPSS Statistics ver. 22.0 (IBM Co., Armonk, NY, USA). Frequency (percentage) was employed to statistically describe count data, and the chi-square test was utilized for statistical analysis. For measures that followed a normal distribution, the mean±standard deviation was reported, with the independent-sample t-test applied for comparisons between groups and the paired-sample t-test for within-group analyses. In cases where measures did not follow a normal distribution, they were presented as median with interquartile range. The Mann-Whitney U-test was used for between-group comparisons, while the Wilcoxon signed-rank test was employed for within-group comparisons. A P-value of less than 0.05 was considered to indicate statistical significance.
RESULTS
In total, 16 HS and 18 UAB patients were recruited in this study. IVES was successful in 11 patients and failed in 7 of the UAB group.
Comparison of General Clinical Data Between the HS and UAB Groups
Table 1 presents the general clinical data for the HS group and the UAB group prior to IVES, while Table 2 details the specific observation indices for patients who experienced successful IVES, both before and after the procedure. There were no significant differences between the HS and UAB groups regarding sex, age, years of education, handedness, or scores for a strong desire to void (P>0.05) (Table 1). However, the UAB group exhibited significantly higher PVR volume, number of 24-hour CIC instances, FS volume, and AUA-SI-QOL scores before IVES compared to the HS group (P<0.001) (Table 1). Additionally, VE was significantly lower in the UAB group (P<0.001) (Table 1). After successful IVES, the PVR, number of 24-hour CIC, FS volume, and AUA-SI-QOL scores significantly decreased (P<0.05) (Table 2), while VE significantly increased (P<0.05) (Table 2).
Comparison of fNIRS Data Between HS and UAB Groups During Voiding
Table 3 presents specific information on changes in significant activation of the PFC during voiding for each group, namely HS, pre- or post-successful IVES, and pre- and post-IVES failure. Initially, no significant activation or deactivation of the PFC was observed in the successful UAB group prior to IVES. However, after IVES, there was notable activation of the right dorsolateral PFC (DLPFC, Ch8) and the right frontal pole (Ch17), as depicted in Fig. 5. Furthermore, BA9 (right DLPFC, Ch8) and BA10 (right frontal pole, Ch1 and Ch11) exhibited significant activation in the successful UAB group post-IVES when compared to their pre-IVES state. Additionally, the level of PFC activation in the successful UAB group after IVES did not significantly differ from that of the HS group (Fig. 5). In contrast, the failed UAB group showed significant deactivation in BA10 (Ch17) and BA46 (right DLPFC, Ch16) before IVES when compared to the HS group (Fig. 6). Specifically, BA10 (Ch17) was significantly deactivated in the failed UAB group relative to the successful UAB group before IVES (Fig. 6).
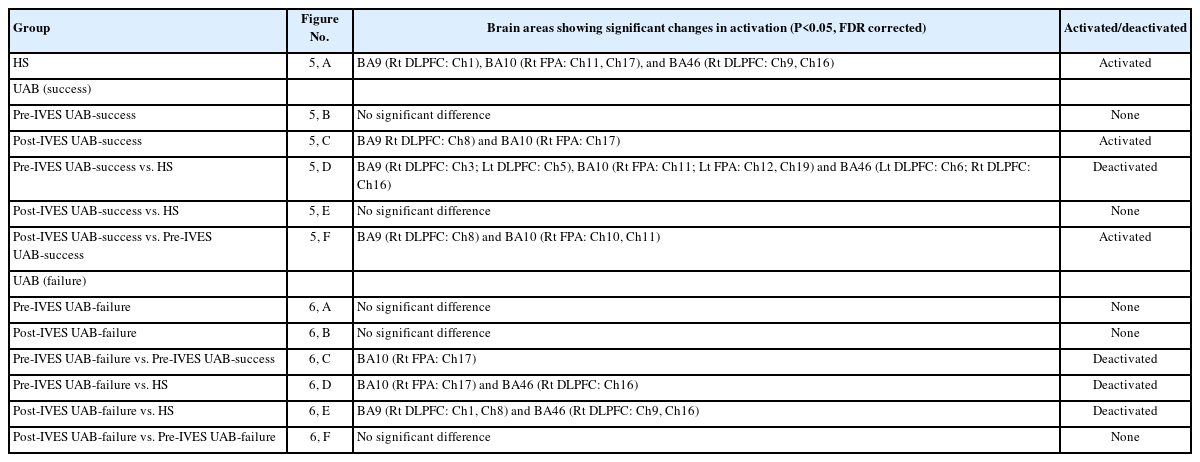
Comparison of fNIRS data of the HS, the successful UAB and the failed UAB groups in the voiding stage

PFC activation changes in the HS and successful UAB groups before and after IVES in the voiding stage. (A) PFC activation in the HS group. (B) PFC activation in the successful UAB group before IVES. (C) PFC activation in the successful UAB group after IVES. (D) PFC activation in the successful UAB group before IVES compared to the HS group. (E) PFC activation in the successful UAB group after IVES compared to the HS group. (F) PFC activation in the successful UAB group after IVES compared to the successful UAB group before IVES. The channels are denoted by dots. Dots marked with red circles represent significant differences for this channel (P<0.05, FDR corrected). The color bars on the right side of the table represent group-level t-values, with warm colors representing activation and cold colors representing deactivation. HS, healthy subject; UAB, underactive bladder; IVES, intravesical electrical stimulation; PFC, prefrontal cortex; FDR, false discovery rate; LH, left hemisphere; RH, right hemisphere.
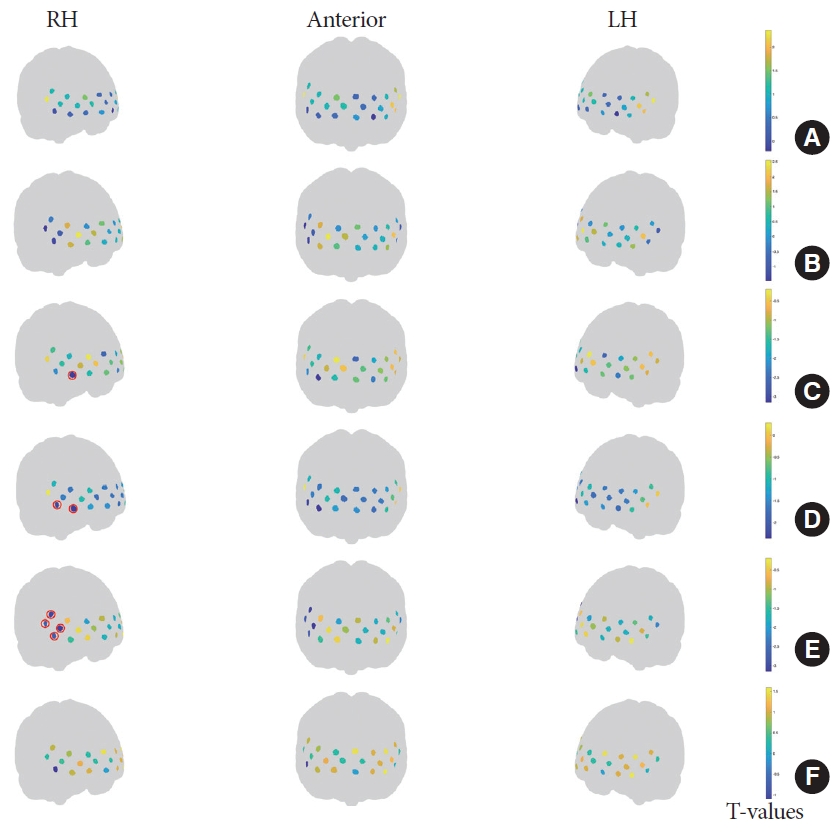
PFC activation changes in the UAB-success and failed UAB groups before and after IVES in the voiding stage. (A) PFC activation in the failed UAB group before IVES. (B) PFC activation in the failed UAB group after IVES. (C) PFC activation in the failed UAB group before IVES compared to the successful UAB group before IVES. (D) PFC activation in the failed UAB group before IVES compared to the HS group. (E) PFC activation in the failed UAB group after IVES compared to the HS group. (F) PFC activation in the failed UAB group after IVES compared to the failed UAB group before IVES. The channels are denoted by dots. Dots marked with red circles represent significant differences for this channel (P<0.05, FDR corrected). The color bars on the right side of the table represent group-level t-values, with warm colors representing activation and cold colors representing deactivation. HS, healthy subject; UAB, underactive bladder; IVES, intravesical electrical stimulation; PFC, prefrontal cortex; FDR, false discovery rate; LH, left hemisphere; RH, right hemisphere.
DISCUSSION
This was the first prospective study to assess the central mechanism of IVES for UAB using fNIRS. Unlike other modalities, fNIRS does not necessitate a specific position for urination and offers vital real-time insights into brain activity changes during voiding. This is crucial for investigating the central brain mechanisms in patients with UAB.
Sakakibara et al. [30] monitored changes in oxy-Hb during natural bladder filling and voiding in healthy adults using fNIRS in real time. They found that the bilateral DLPFC was significantly activated during these processes, with oxy-Hb concentrations in the bilateral DLPFC returning to baseline after voiding. This indicates that the bilateral DLPFC plays a crucial role in the brain-bladder control mechanism for urination. Activation and deactivation in the frontal cortex correspond to increases and decreases in oxy-Hb concentration and cerebral blood flow, respectively. In our study, we observed no significant changes in PFC activation or deactivation in the successful UAB group before IVES. However, after IVES, there was significant activation in BA9 and BA10, indicating that IVES markedly increased local cerebral blood flow to the right DLPFC and right frontal pole during voiding. Additionally, we noted significant activation in BA9, BA10, and BA46 (right DLPFC) in the HS group during voiding. This finding implies that the right DLPFC and right frontal pole are engaged in the brain-bladder process of urinary control and are activated during normal urination. We also discovered that BA9 and BA10 showed significant activation in the successful UAB group after IVES compared to before the treatment, with no significant difference in PFC activation levels between the successful UAB group and the HS group post-IVES. These results suggest that IVES may have normalized the brain activity in the right DLPFC and right frontal pole of UAB patients, indicating that neuroplasticity could be a key factor in the success of IVES in treating UAB.
Matsumoto et al. [31] reported that in a study of healthy adults using fNIRS, increased sensations of bladder voiding were associated with elevated oxy-Hb levels in the bilateral frontal cortex. Similarly, significant activation of the bilateral DLPFC during natural bladder filling and voiding has been documented [30]. However, Sakakibara et al. [32] posited that the right frontal region plays a crucial role in voiding regulation, with the right hemisphere being predominantly involved. In our study, we observed significant activation exclusively in the right PFC during voiding among both healthy adults and UAB patients who responded to IVES treatment. This finding may corroborate the right hemisphere’s dominance in the voiding process.
It has been suggested that reduced local blood flow in the PFC is linked to impaired bladder sensation [33]. Furthermore, the PFC is implicated in sensory integration, cognitive processing, and decision-making [34,35]. In our study, patients in the successful UAB group exhibited a significant increase in FS volume following IVES treatment. This suggests that IVES may exert its therapeutic effects by improving abnormal bladder afferent signaling through the modulation of ascending sensory nerve impulses.
Before IVES, BA10 was significantly less active in the failed UAB group than in the successful UAB group. Upon comparing the failed UAB group with the HS group post-IVES treatment, we observed that the significant reduction in activity persisted in the failed UAB treatment group. Consequently, we propose that a higher level of deactivation in the right frontal pole may be associated with an unfavorable IVES outcome, a hypothesis that warrants verification by increasing the sample size.
The limitations of this study are as follows: (1) Due to the technical constraints of fNIRS, we were unable to detect the deep nuclei of the brain. In future research, we plan to enhance the depth of detection by integrating additional technical methods. (2) We only monitored PFC function using 22 channels, leaving functional changes in other brain regions unexplored. (3) Patients underwent just one fNIRS scan after receiving 4 weeks of IVES treatment. Future studies should include fNIRS scans after more extended treatment periods and long-term follow-up to assess the persistence of treatment effects. (4) The study’s sample size was limited, and to standardize the voiding position, we included only female subjects. In subsequent research, we aim to recruit a larger, more diverse cohort that includes male participants to further investigate the mechanism of action of IVES.
In conclusion, the central mechanism by which IVES treatment benefits patients with neurogenic UAB could be the reactivation of the DLPFC and the right frontal pole. Our findings shed light on the central mechanism of IVES and could inform the development of future treatments.
Notes
Grant/Fund Support
This study was funded by the Capital’s Funds for Health Improvement and Research (No. 2022-1-6011), Fundamental Research Funds for Central Public Welfare Research Institutes (No. 2023CZ-1), and Beijing Natural Science Foundation of China (No. 7202236).
Research Ethics
This study was reviewed and approved by the Ethics Committee of China Rehabilitation Research Center (IRB: 2021-085-1). All participants provided written informed consent to participate in this study.
Conflict of Interest
No potential conflict of interest relevant to this article was reported.
AUTHOR CONTRIBUTION STATEMENT
· Conceptualization: HD
· Data curation: LW
· Formal analysis: JW, LW
· Funding acquisition: LL
· Methodology: HD, LL
· Project administration: HD
· Visualization: HD
· Writing - original draft: HD
· Writing - review & editing: HD, LL, JW